by Carrie Decker, ND
Many clinicians may shy away from treating Lyme disease patients. This may be because very few had an immersed internship, or they knew, likely from a personal or close family member’s experience, that working with Lyme disease was a personal passion they would pursue until unable any more. So, how do we step into the world of Lyme and work with this patient population and all its complications? An understanding of the challenges that can make this infectious disease so chronic helps us to recognize what tools from our holistic toolbox may be useful in not only the eradication of this infection, but also for palliating the symptoms with which our patients may suffer.
Lyme disease specifically refers to the tick-borne infection typically caused by one of three pathogenic species of the spirochete Borrelia burgdorferi sensu lato.1 Of these, in the United States, B. burgdorferi is the most common, while B. afzelii and B. garinii have been observed more in Europe as well as Asia. Many new species and variants within this family continue to be recognized.2 However, B. burgdorferi, or one of these others, is not the only pathogenic agent which a tick may transmit. Common co-infections include microbes from the families of Bartonella, Ehrlichia, Anaplasma, and Babesia,3,4 which not only lead to symptoms associated with their presence but also can increase Lyme disease severity and have their own distinct challenges in the process of eradication.5 The various diagnostic tests on the marketplace, and their advantages or pitfalls, are beyond the scope of this article and are a complex discussion as well.
The Borrelia spirochete not only often travels with a companion, but itself can take many forms in the body. It is able to defend itself by switching to a cystic form or existing within a biofilm-like colony, as well as by differentially expressing proteins and genes that enable survival in the host.6-8 By doing such, it is not only able to avoid immune system clearance, it also is able to avoid detection.9,10 Because of this switching, we will see not only resistance to antibiotics, but also botanicals, necessitating an ongoing process of switching our therapies, often through a long course of treatment.
Given these issues, the interventions selected for the treatment of Lyme disease must consider the broad array of pathogens that may exist, the protective nature of biofilms that prevent eradication, and support to encourage a necessary, yet balanced, immune system response. With the vast array of symptoms which are debilitating for many, support for symptom palliation, ideally in a manner that does not suppress but rather further encourages healing, also is necessary.
Antimicrobial Therapies
Artemisia annua, also known as sweet wormwood, or Qinghao, has a long history of use as an antimicrobial agent.11,12 The primary compound derived from this plant is artemisinin, also known as Qinghaosu.13 However, additional bioactive substances found in its oil include camphor, germacrene D, artemisia ketone and 1,8 cineole.14
A. annua has broad-spectrum action as an antiparasitic, antibacterial, antifungal, and antiviral agent. A. annua and its derivatives, particularly artemisinin, have been shown to be active against Babesia, as well as cytomegalovirus, herpes simplex virus, Epstein-Barr virus, and Toxoplasmosis gondii – each of these being additional common causes of persistent, chronic infections that burden the immune system.15-18 Action has also been demonstrated against Staphylococcus aureus, Streptococcus pneumoniae, Escherichia coli, E. coli UPEC, Haemophilus influenzae, Helicobacter pylori, Pseudomonas aeruginosa, Campylobacter jejuni, Clostridium perfringens, and Candida spp.,19-22 many of which may exist as pathogens or at unbalanced levels in the often immune-compromised Lyme patient. Artemisinin also may impact biofilms, which make some of these pathogens resistant to treatment.23,24
In a recent study of patients experiencing short-term memory deficits associated with Lyme disease, oral treatment with artesunate, a water-soluble artemisinin derivative, was shown to significantly reduce the short-term memory difficulties.25 Although the memory issues may occur due to central nervous system infection with Borrelia spp., this symptom also may be attributable to a Babesia co-infection. Regardless of the infectious etiology, this recent finding is noteworthy and may translate to clinical improvements.
Grapefruit seed extract, although the subject of some controversy due to the possible contamination of products with benzethonium chloride and triclosan,26,27 also may be helpful in the treatment of Lyme disease, due to its demonstrated action against both the motile and cystic forms of B. burgdorferi.28,29 It has been reported to have no significant adverse effects, including on the population of healthy Lactobacillus spp. or Bifidobacterium spp. in the gut.30
Immune Support
Many botanicals have evidence of supporting immune system function; of these, one which is of importance in the treatment of Lyme disease is cat’s claw (Uncaria tomentosa), also known in Spanish as uña de gato. Cat’s claw is ideally suited in the Lyme setting, as in addition to its immune-supportive actions, it has been shown to have anti-inflammatory, anti-arthritic, and antioxidant effects, and supports cognitive function. Cat’s claw has been shown to enhance proliferation of both T helper and B lymphocytes,31,32 also increasing lymphocyte viability and survival. In the setting of rheumatoid arthritis, cat’s claw has been shown to significantly reduce the number of painful and swollen joints.33 In osteoarthritis, similar benefits have been seen, with a significant improvement in pain associated with activity, as well as medical and patient symptom assessment scores.34 Potent antioxidant activity, including the protection of membrane lipids from peroxidation, has been demonstrated in multiple in vitro studies, along with a strong level of inhibition of tumor necrosis factor (TNF)-α, a primary pro-inflammatory cytokine associated with the acute immune system response.35,36
Cat’s claw also has the potential to improve some of the cognitive symptoms seen with Lyme disease and its common co-infections. In multiple animal models, cat’s claw has been demonstrated to have a neuroprotective effect and improve memory,37,38 possibly attributable to its antioxidant action or altered glutaminergic signaling.39
Reducing Biofilms and Taming the Inflammatory Response
Lactoferrin, a glycoprotein found in milk and at much higher concentrations in colostrum, has long been recognized for the role it plays in protecting infants from infection as well as supporting normal immune system function.40 It has been shown to have antimicrobial activity against parasites, bacteria, fungi, and viruses,41 and supports the body’s protective response to tick-borne pathogens. Lactoferrin has been shown to have an inhibitory effect on bacterial biofilms,42 including that of B. burgdorferi.43 Lactoferrin also has been shown to inhibit the growth of Babesia spp., one of the common co-infections.44
Lactoferrin’s immunomodulating effects support symptom reduction and an improved systemic response. Many Lyme patients not only experience symptoms due to the active infection in their body; but when treatment commences, a reaction referred to as “die-off,” or formally, a Jarisch-Herxheimer reaction, may occur. Specifically, a Jarisch-Herxheimer reaction is the symptoms that transpire due to the uncontrolled release of endotoxin (also known as lipopolysaccharide or LPS), as well as endotoxin-like products, during lysis of the broad spectrum of bacteria which many natural and pharmaceutical antibiotics impact.45 These products of bacterial die-off not only stimulate a further immune response and inflammation, they also can adversely affect organ and systemic function, sometimes critically, in patients with Lyme disease.46,47 Lactoferrin has been shown to neutralize endotoxin,48 also directly inhibiting the endotoxin-induced immune system response.49,50 Lactoferrin has also been shown in several studies to decrease levels of TNF-α.51 In animal models, the administration of supplemental lactoferrin prior to endotoxin shock dramatically reduced mortality and increased overall wellness.52,53
Chitosan is a biopolymer derived from chitin, a component of the shell of crustaceans that is used in a variety of biological applications including as a vaccine adjuvant. Chitosan also is used in an array of applications as a chelator.54,55 It has been shown to have the ability to bind and remove a variety of toxins including polychlorinated biphenyls, phthalates, bisphenol A, mold toxins, and heavy metals.56-59 It also has an ability to chelate and remove the heavy metals manganese and zinc.60,61 Manganese and zinc are two minerals that are essential for the lifecycle and metabolic needs of B. burgdorferi, also serving as central regulators of many of its virulence genes.62,63
Chitosan has been shown to have an antimicrobial effect via disrupting biofilms associated with Streptococcus mutans and C. albicans, particularly when the chitosan is of low molecular weight.64,65 Chitosan also has the ability to bind endotoxin 66,67 and has been shown specifically, in the setting of Lyme, to reduce symptoms attributed to the Jarisch-Herxheimer reaction.68 In the gut, chitosan has been shown to have a prebiotic effect, promoting the growth of Bifidobacterium spp. and Lactobacillus spp., which are predominant healthy flora that also support the reduction of inflammation and a normal immune response.69-71
Support for Cellular Function
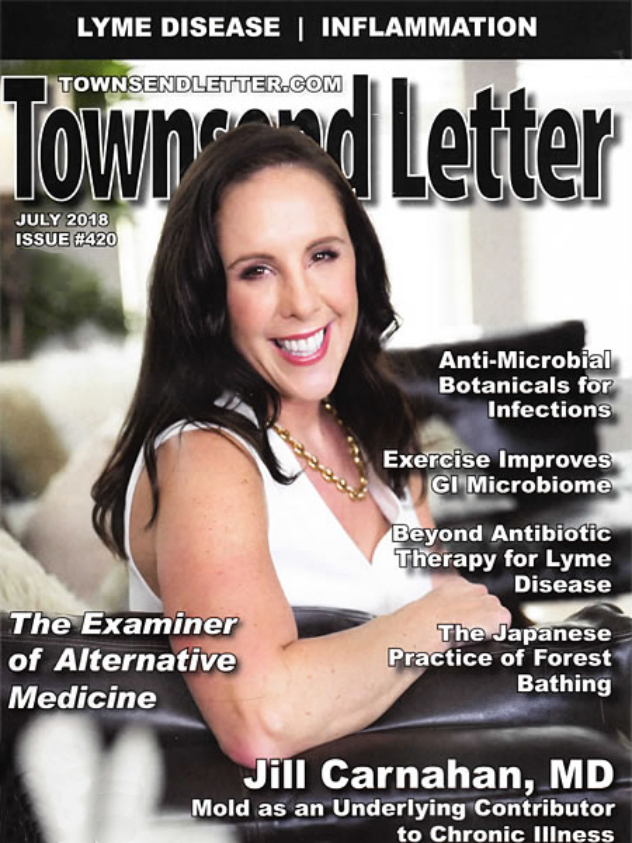
One possible contributor to the fatigue that is experienced with Lyme disease, and with other chronic infections for that matter, is altered cellular function due to oxidative stress and damage. In Lyme borreliosis patients, classified by the CDC definition of Lyme disease and not being treated by antibiotics, significantly low levels of cytosolic ionized calcium, indicative of interrupted cellular communication, and significantly higher levels of mitochondrial superoxide, indicative of oxidative stress and damage within the cell, have been shown.72 Additionally, B. burgdorferi has been shown to sequester cysteine, the rate-limiting amino acid for glutathione production, for its growth.73 This may further impair cellular function and detoxification, leaving the cell even more susceptible to oxidative damage particularly if additional insults occur. It is not uncommon that we see patients with Lyme disease easily fall ill when exposed to mold or heavy metals; the reduced ability for the cells to detoxify and carry on normal function may be a significant contributor.
Lipid replacement therapy, that is, oral supplementation with glycerolphospholipids, which are the main component of cellular membranes, is one strategy to support normal cellular function and repair. To further enhance this, critical antioxidants such as coenzyme Q10 (CoQ10) and reduced nicotinamide adenine dinucleotide (NADH) are also often provided as well. The combination therapy of glycerolphospholipids, CoQ10, NADH, and additional ingredients to support cellular function, such as L-carnitine, has been studied in the setting of intractable chronic fatigue and chronic Lyme disease, diagnosed by symptoms and positive Western blog analysis. After two months of daily supplemental support, fatigue, as well as all subcategories of fatigue including cognitive function and mood, were significantly improved (P<0.0001).74 Trendlines also showed significant and consistent downward trends in the data, indicating that symptoms would have further improved with time. Additional studies utilizing glycerolphospholipids as a monotherapy or in combination with additional nutrients have shown positive outcomes in similar settings of chronic fatigue, also leading to improvement in mitochondrial function.75,76
Although the challenges associated with supporting patients whose health has been compromised by a tick-borne infection do have many complexities, therapies such as these, or a combination thereof, offer a starting point that clinicians can trust, due to evidence in in vitro, animal, and human clinical studies that support their use. Other botanical and nutritional substances, as well as energetic medicine and physical modalities, also are often necessary for complete recovery; but for some, therapies such as these discussed herein may be adequate to encourage the body to respond and recover.
Carrie Decker is a certified naturopathic doctor, graduating with honors from the National College of Natural Medicine (now the National University of Natural Medicine) in Portland, Oregon. Dr. Decker sees patients at her office in Portland, Oregon, as well as remotely, with a focus on gastrointestinal disease, mood imbalances, eating disorders, autoimmune disease, and chronic fatigue. Prior to becoming a naturopathic physician, Dr. Decker was an engineer and obtained graduate degrees in biomedical and mechanical engineering from the University of Wisconsin-Madison and University of Illinois at Urbana-Champaign respectively. Dr. Decker continues to enjoy academic research and writing and uses these skills to support integrative medicine education as a writer and contributor to various resources. Dr. Decker supports Allergy Research Group as a member of their education and product development team.
References
1. Balmelli T, Piffaretti JC. Association between different clinical manifestations of Lyme disease and different species of Borrelia burgdorferi sensu lato. Res Microbiol. 1995 May;146(4):329-40.
2. Rudenko N, et al. Updates on Borrelia burgdorferi sensu lato complex with respect to public health. Ticks Tick Borne Dis. 2011 Sep;2(3):123-8.
3. Lantos PM, Wormser GP. Chronic coinfections in patients diagnosed with chronic lyme disease: a systematic review. Am J Med. 2014 Nov;127(11):1105-10.
4. Mitchell PD, et al. Immunoserologic evidence of coinfection with Borrelia burgdorferi, Babesia microti, and human granulocytic Ehrlichia species in residents of Wisconsin and Minnesota. J Clin Microbiol. 1996 Mar;34(3):724-7.
5. Diuk-Wasser MA, et al. Coinfection by Ixodes Tick-Borne Pathogens: Ecological, Epidemiological, and Clinical Consequences. Trends Parasitol. 2016 Jan;32(1):30-42.
6. Zajkowska J, et al. [Atypical forms of Borrelia burgdorferi–clinical consequences]. Pol Merkur Lekarski. 2005 Jan;18(103):115- 9.
7. Singh SK, Girschick HJ. Molecular survival strategies of the Lyme disease spirochete Borrelia burgdorferi. Lancet Infect Dis. 2004 Sep;4(9):575-83.
8. Sapi E, et al Characterization of biofilm formation by Borrelia burgdorferi in vitro. PloS One. 2012 Oct 24;7(10):e48277.
9. Miklossy J, et al. Persisting atypical and cystic forms of Borrelia burgdorferi and local inflammation in Lyme neuroborreliosis. J Neuroinflammation. 2008 Sep 25;5:40.
10. Steere AC, et al. Prospective study of serologic tests for lyme disease. Clin Infect Dis. 2008 Jul 15;47(2):188-95.
11. Klayman DL. Qinghaosu (artemisinin): an antimalarial drug from China. Science. 1985 May 31;228(4703):1049-55.
12. Tariq A, et al. Ethnomedicines and anti-parasitic activities of Pakistani medicinal plants against Plasmodia and Leishmania parasites. Ann Clin Microbiol Antimicrob. 2016 Sep 20;15(1):52.
13. White NJ, et al. A Brief History of Qinghaosu. Trends Parasitol. 2015 Dec;31(12):607-610.
14. Bilia AR, et al. Essential Oil of Artemisia annua L.: An Extraordinary Component with Numerous Antimicrobial Properties. Evid Based Complement Alternat Med. 2014;2014:159819.
15. Efferth T, et al. The antiviral activities of artemisinin and artesunate. Clin Infect Dis. 2008 Sep 15;47(6):804-11.
16. D’Angelo JG, et al. Artemisinin derivatives inhibit Toxoplasma gondii in vitro at multiple steps in the lytic cycle. J Antimicrob Chemother. 2009 Jan;63(1):146-50.
17. Loo CS, et al. Artemisinin and its derivatives in treating protozoan infections beyond malaria. Pharmacol Res. 2017 Mar;117:192-217.
18. Goo YK, et al. Artesunate, a potential drug for treatment of Babesia infection. Parasitol Int. 2010 Sep;59(3):481-6.
19. Goswami S, et al. Anti-Helicobacter pylori potential of artemisinin and its derivatives. Antimicrob Agents Chemother. 2012 Sep;56(9):4594-607.
20. Bilia AR, et al. Essential Oil of Artemisia annua L.: An Extraordinary Component with Numerous Antimicrobial Properties. Evid Based Complement Alternat Med. 2014;2014:159819.
21. Militaru D, et al. In vitro evaluation of the potential antibacterial effect of artemisinin on Campylobacter jejuni. Rom Biotech Let. 2015 Mar 1;20(2):10221-7.
22. Engberg RM, et al. The effect of Artemisia annua on broiler performance, on intestinal microbiota and on the course of a Clostridium perfringens infection applying a necrotic enteritis disease model. Avian Pathology. 2012 Aug 1;41(4):369-76.
23. De Cremer K, et al. Artemisinins, new miconazole potentiators resulting in increased activity against Candida albicans biofilms. Antimicrob Agents Chemother. 2015 Jan;59(1):421-6.
24. Sisto F, et al. In vitro activity of artemisone and artemisinin derivatives against extracellular and intracellular Helicobacter pylori. Int J Antimicrob Agents. 2016 Jul;48(1):101-5
25. Puri BK, et al. The effect of artesunate on short-term memory in Lyme borreliosis. Med Hypotheses. 2017 Aug;105:4-5.
26. Avula B, et al. Simultaneous identification by liquid chromatography of benzethonium chloride, methyl paraben and triclosan in commercial products labeled as grapefruit seed extract. Pharmazie. 2007 Aug;62(8):593-596.
27. Sugimoto N, et al. [Survey of synthetic disinfectants in grapefruit seed extract and its compounded products]. Shokuhin Eiseigaku Zasshi. 2008 Feb;49(1):56-62.
28. Brorson O, Brorson SH. Grapefruit seed extract is a powerful in vitro agent against motile and cystic forms of Borrelia burgdorferi sensu lato. Infection. 2007 Jun 1;35(3):206.
29. Goc A, Rath M. The anti-borreliae efficacy of phytochemicals and micronutrients: an update. Ther Adv Infect Dis. 2016 Jun;3(3- 4):75-82.
30. Ionescu G, et al. Oral citrus seed extract in atopic eczema: In vitro and in vivo studies on intestinal microflora. J Orthomolecular Med. 1990;5:155-7.
31. Wurm M, et al. Pentacyclic oxindole alkaloids from Uncaria tomentosa induce human endothelial cells to release a lymphocyteproliferation-regulating factor. Planta Med. 1998 Dec;64(8):701-4.
32. Domingues A, et al. Uncaria tomentosa aqueous-ethanol extract triggers an immunomodulation toward a Th2 cytokine profile. Phytother Res. 2011 Aug;25(8):1229-35.
33. Mur E, et al. Randomized double blind trial of an extract from the pentacyclic alkaloid-chemotype of uncaria tomentosa for the treatment of rheumatoid arthritis. J Rheumatol. 2002 Apr;29(4):678-81.
34. Piscoya J, et al. Efficacy and safety of freeze-dried cat’s claw in osteoarthritis of the knee: mechanisms of action of the species Uncaria guianensis. Inflamm Res. 2001 Sep;50(9):442-8.
35. Gonçalves C, Dinis T, Batista MT. Antioxidant properties of proanthocyanidins of Uncaria tomentosa bark decoction: a mechanism for anti-inflammatory activity. Phytochemistry. 2005 Jan;66(1):89-98.
36. Sandoval M, et al. Cat’s claw inhibits TNFalpha production and scavenges free radicals: role in cytoprotection. Free Radic Biol Med. 2000 Jul 1;29(1):71-8.
37. Mohamed AF, et al. Effects of Uncaria tomentosa total alkaloid and its components on experimental amnesia in mice: elucidation using the passive avoidance test. J Pharm Pharmacol. 2000 Dec;52(12):1553-61.
38. Lee SC, et al. Effects of repeated administration of Uncaria hooks on the acquisition and central neuronal activities in ethanoltreated mice. J Ethnopharmacol. 2004 Sep;94(1):123-8.
39. Santo GD, et al. Protective effect of Uncaria tomentosa extract against oxidative stress and genotoxicity induced by glyphosateRoundup® using zebrafish (Danio rerio) as a model. Environ Sci Pollut Res Int. 2018 Feb 13.
40. Siqueiros-Cendón T, et al. Immunomodulatory effects of lactoferrin. Acta Pharmacol Sin. 2014 May;35(5):557-66.
41. Moreno-Expósito L, et al. Multifunctional capacity and therapeutic potential of lactoferrin. Life Sci. 2018 Feb 15;195:61-64.
42. Singh PK, et al. A component of innate immunity prevents bacterial biofilm development. Nature. 2002 May;417(6888):552.
43. Haenel D, Sapi E. Significant antimicrobial effects of lactoferrin on Borrelia burgdorferi biofilm. University of New Haven. Poster.
44. Ikadai H, et al. Inhibitory effect of lactoferrin on in vitro growth of Babesia caballi. Am J Trop Med Hyg. 2005 Oct;73(4):710-2.
45. Butler T. The Jarisch-Herxheimer Reaction After Antibiotic Treatment of Spirochetal Infections: A Review of Recent Cases and Our Understanding of Pathogenesis. Am J Trop Med Hyg. 2017 Jan 11;96(1):46-52.
46. Maloy AL, Black RD, Segurola RJ Jr. Lyme disease complicated by the Jarisch-Herxheimer reaction. J Emerg Med. 1998 MayJun;16(3):437-8.
47. Webster G, et al. Jarisch-Herxheimer reaction associated with ciprofloxacin administration for tick-borne relapsing fever. Pediatr Infect Dis J. 2002 Jun;21(6):571-3.
48. Zhang GH, et al. Neutralization of endotoxin in vitro and in vivo by a human lactoferrin-derived peptide. Infect Immun. 1999 Mar;67(3):1353-8.
49. Elass-Rochard E, et al. Lactoferrin inhibits the endotoxin interaction with CD14 by competition with the lipopolysaccharidebinding protein. Infect Immun. 1998 Feb;66(2):486-91.
50. Mattsby-Baltzer I, et al. Lactoferrin or a fragment thereof inhibits the endotoxin-induced interleukin-6 response in human monocytic cells. Pediatr Res. 1996 Aug;40(2):257-62.
51. Drago-Serrano ME, et al. Lactoferrin: Balancing Ups and Downs of Inflammation Due to Microbial Infections. Int J Mol Sci. 2017 Mar 1;18(3).
52. Kruzel ML, et al. Lactoferrin protects gut mucosal integrity during endotoxemia induced by lipopolysaccharide in mice. Inflammation. 2000 Feb;24(1):33-44.
53. Lee WJ, et al. The protective effects of lactoferrin feeding against endotoxin lethal shock in germfree piglets. Infect Immun. 1998 Apr;66(4):1421-6.
54. Gerente C, et al. Application of chitosan for the removal of metals from wastewaters by adsorption—mechanisms and models review. Crit Rev Enviro Sci Tech. 2007 Jan 1;37(1):41-127.
55. Dehghani MH, et al. Adsorptive removal of endocrine disrupting bisphenol A from aqueous solution using chitosan. J Enviro Chem Eng. 2016 Sep 30;4(3):2647-55.
56. Salim CJ, Liu H, Kennedy JF. Comparative study of the adsorption on chitosan beads of phthalate esters and their degradation products. Carbo Polymers. 2010 Jul 7;81(3):640-4.
57. Dehghani MH, et al. Adsorptive removal of endocrine disrupting bisphenol A from aqueous solution using chitosan. J Enviro Chem Eng. 2016 Sep 30;4(3):2647-55.
58. Bornet A, Teissedre PL. Chitosan, chitin-glucan and chitin effects on minerals (iron, lead, cadmium) and organic (ochratoxin A) contaminants in wines. Euro Food Res Tech. 2008 Feb 1;226(4):681-9.
59. Quintela S, et al. Ochratoxin A removal from red wine by several oenological fining agents: bentonite, egg albumin, allergenfree adsorbents, chitin and chitosan. Food Addit Contam Part A Chem Anal Control Expo Risk Assess. 2012;29(7):1168-74.
60. Guan B, et al. Removal of Mn (II) and Zn (II) ions from flue gas desulfurization wastewater with water-soluble chitosan. Sep Purif Tech. 2009 Mar 12;65(3):269-74.
61. Wu ZB, Ni WM, Guan BH. Application of chitosan as flocculant for coprecipitation of Mn (II) and suspended solids from dualalkali FGD regenerating process. J Haz Mat. 2008 Apr 1;152(2):757-64.
62. Troxell B, et al. Manganese and zinc regulate virulence determinants in Borrelia burgdorferi. Infect Immun. 2013 Aug;81(8):2743-52.
63. Aguirre JD, et al. A manganese-rich environment supports superoxide dismutase activity in a Lyme disease pathogen, Borrelia burgdorferi. J Biol Chem. 2013 Mar 22;288(12):8468-78.
64. Pu Y, et al. In vitro damage of Candida albicans biofilms by chitosan. Exp Ther Med. 2014 Sep;8(3):929-934.
65. Chávez de Paz LE, et al. Antimicrobial effect of chitosan nanoparticles on streptococcus mutans biofilms. Appl Environ Microbiol. 2011 Jun;77(11):3892-5.
66. Davydova VN, et al. Interaction of bacterial endotoxins with chitosan. Effect of endotoxin structure, chitosan molecular mass, and ionic strength of the solution on the formation of the complex. Biochemistry (Mosc). 2000 Sep;65(9):1082-90.
67. Solov’eva T, et al. Marine compounds with therapeutic potential in gram-negative sepsis. Mar Drugs. 2013 Jun 19;11(6):2216- 29.
68. Hines SW. Nano-Particle Chitosan: New Hope for Lyme-Related Herxheimer Symptoms. Focus. 2007 July:9-10.
69. Lee HW, et al. Chitosan oligosaccharides, dp 2-8, have prebiotic effect on the Bifidobacterium bifidium and Lactobacillus sp. Anaerobe. 2002 Dec;8(6):319-24.
70. Cani PD, et al. Selective increases of bifidobacteria in gut microflora improve high-fat-diet-induced diabetes in mice through a mechanism associated with endotoxaemia. Diabetologia. 2007 Nov 1;50(11):2374-83.
71. Roselli M, et al. Probiotic bacteria Bifidobacterium animalis MB5 and Lactobacillus rhamnosus GG protect intestinal Caco-2 cells from the inflammation-associated response induced by enterotoxigenic Escherichia coli K88. Brit J Nutr. 2006 Jun;95(6):1177-84.
72. Peacock BN, et al. New insights into Lyme disease. Redox Biol. 2015 Aug;5:66-70.
73. Sambri V, Cevenini R. Incorporation of cysteine by Borrelia burgdorferi and Borrelia hermsii. Can J Microbiol. 1992 Oct;38(10):1016-21.
74. Nicolson GL, et al. Lipid replacement therapy with a glycophospholipid formulation with NADH and CoQ10 significantly reduces fatigue in intractable chronic fatiguing illnesses and chronic Lyme disease patients. Int J Clin Med. 2012 May 29;3(03):163.
75. Nicolson GL. Lipid replacement/antioxidant therapy as an adjunct supplement to reduce the adverse effects of cancer therapy and restore mitochondrial function. Pathol Oncol Res. 2005;11(3):139-44.
76. Agadjanyan M, et al. Nutritional supplement (NT Factor™) restores mitochondrial function and reduces moderately severe fatigue in aged subjects. J Chronic Fat Syn. 2003;11(3):23-36.